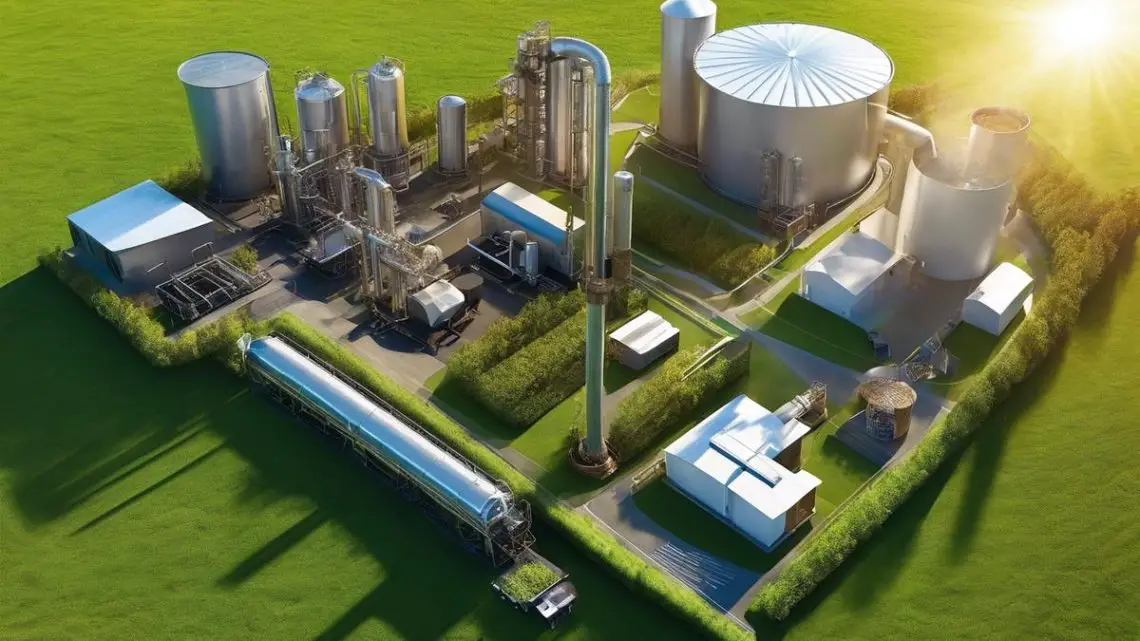
Biomass Energy: Pros and Cons Explained
January 19, 2024As the quest for sustainable energy intensifies, biomass emerges as a pivotal player in the arena of renewable resources. This exploration into the realm of biomass energy untangles the complexities and delves into its multifaceted nature, uncovering the potential of plant-based and waste-derived materials as alternative sources of power.
Through the lens of environmental stewardship and innovation, this journey will dissect the layers of organic materials that form the backbone of biomass energy, venturing beyond the surface to examine the environmental, economic, and social narratives that intertwine with the fabric of this energy form. As we navigate the intricacies of biomass conversion processes and their impacts on our planet, we encroach upon a dialogue pivotal for the present and future of energy consumption.
Types of Biomass Materials and Conversion Processes
Unearthing Energy: The Conversion of Biomass Resources
How does biomass energy work? Biomass energy harnesses organic matter, including wood, agricultural crops, and waste by-products, as fuel. It’s a renewable resource that’s widely available in nature. As we seek alternatives to fossil fuels, biomass holds significant potential due to its sustainability. The primary sources of biomass are forest residues like branches and stumps from timber harvests (woody biomass), plant materials such as corn leaves and stalks (agricultural biomass), and waste products from municipal and industrial processes (waste biomass).
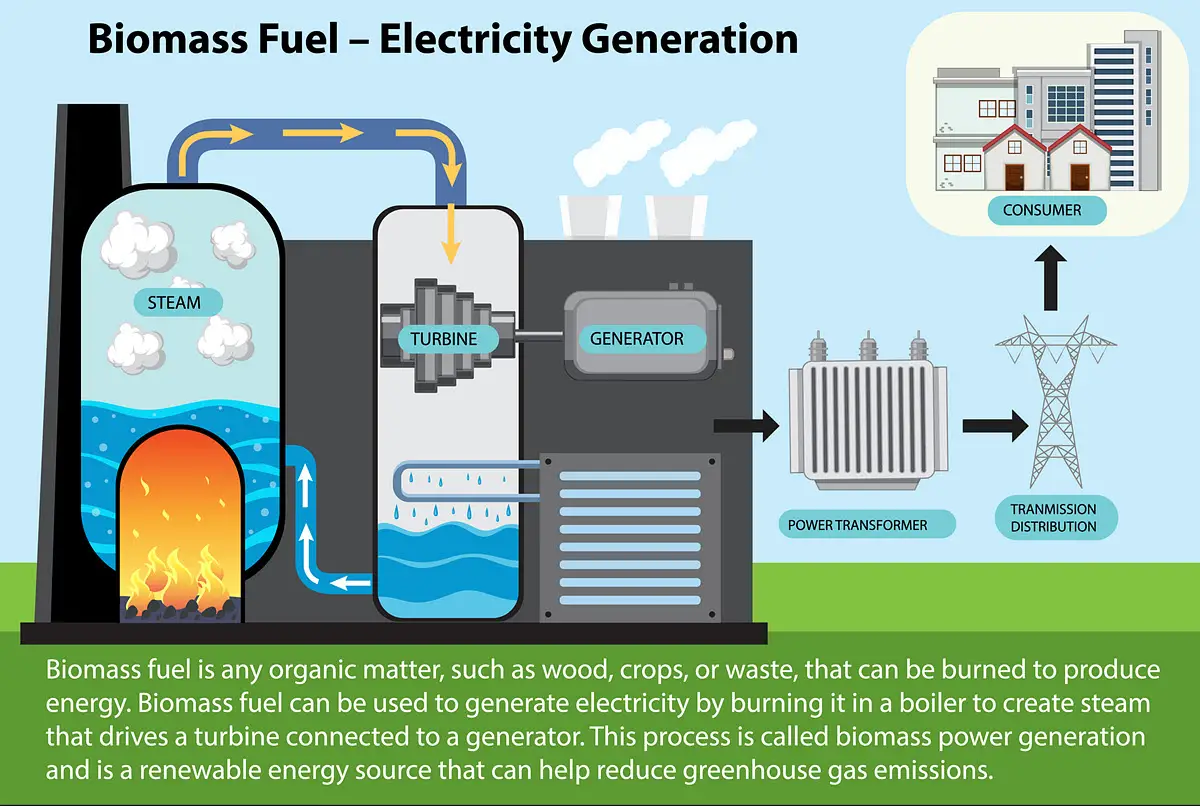
Credit: Photo by depositphotos.com
The transformation of these organic substances into usable energy forms encompasses several techniques, namely combustion, gasification, pyrolysis, and anaerobic digestion.
-
- Combustion
The most direct method is combustion, where biomass is burned to heat water in a boiler, generating steam. This steam runs through turbines, propelling generators to create electricity. Commonly, woody biomass is utilized in combustion processes at large-scale facilities, providing a steady source of renewable energy.
-
- Gasification
Gasification involves subjecting biomass to high temperatures in an environment with controlled oxygen, which converts the material into a mixture of gases including hydrogen, carbon monoxide, and methane, collectively known as syngas. Syngas can be subsequently burned to generate electricity or processed into transportation fuels.
-
- Pyrolysis
Pyrolysis heats biomass in the absence of oxygen, breaking it down into a liquid called bio-oil, alongside small amounts of gas and char. Bio-oil can be upgraded to fuels or used directly for heat, while the gas can be harnessed for energy production.
-
- Anaerobic Digestion
Anaerobic digestion occurs in an oxygen-free environment where organic materials, particularly waste biomass like manure and wastewater solids, are decomposed by microorganisms. This process yields biogas, predominantly composed of methane and carbon dioxide, which can be used as a fuel for heating, electricity generation, or as a natural gas alternative.
Environmental Impact of Biomass Energy
The environmental impacts resulting from the production of biomass energy are multifaceted, posing both benefits and dilemmas. A cogent understanding of these effects is imperative to discern the sustainability of biomass as an alternative to fossil fuels.
Foremost, biomass energy production necessitates the utilization of organic materials, which, when compared to fossil fuels, emit lower levels of carbon dioxide—an influential greenhouse gas—when combusted or digested. This ostensibly reduced carbon footprint is predicated on the notion that the carbon released during biomass energy production is part of a cyclical process, whereby carbon dioxide is absorbed by plant growth in a relatively short time frame, thus theoretically achieving a measure of carbon neutrality.
Cons of Biomass Energy
Nonetheless, this perspective does not account for the entire ecological narrative. The cultivation of biomass can lead to alterations in land use, occasionally resulting in the depletion of valuable ecosystems, including forests and grasslands, which in turn jeopardizes biodiversity. Moreover, such cultivation practices often require substantial amounts of water and fertilizers, potentially imposing strain on water resources and contributing to nutrient runoff, which can eutrophy water bodies and disrupt aquatic ecosystems.
Equally important is the consideration of air quality. Despite lower carbon dioxide emissions, the combustion of biomass can release other pollutants, including particulate matter, nitrogen oxides, and volatile organic compounds, which may affect local air quality and raise health concerns. Ensuring that combustion technologies adhere to stringent emission standards is crucial to minimizing such detrimental impacts.
The management of waste products from biomass energy processes also holds significant environmental implications. For instance, ash resulting from combustion necessitates careful handling to prevent soil and water contamination. Meanwhile, the production of biochar—a byproduct of pyrolysis—presents a beneficial opportunity to sequester carbon and enhance soil fertility, provided its application is executed judiciously.
In pursuit of environmental amelioration, life cycle assessments (LCAs) are employed to evaluate the comprehensive environmental impacts of biomass energy production. By examining everything from the sourcing of raw materials to the eventual disposal or recycling of waste, LCAs can guide the refinement of biomass technologies and practices, aiming to minimize harmful consequences while optimizing energy yields.
In the endeavor to reconcile the energy demands of an ever-expanding human population with the exigencies of ecological stewardship, an intricate balance must be sustained. The landscape of biomass energy production, then, is one of constant exploration, necessitating rigorous scientific scrutiny, innovative technological solutions, and a judicious approach to resource management. While the utilitarian aspects of biomass are clear, vigilance and adaptability remain paramount to ensure that its deployment augments, rather than diminishes, environmental integrity.
Economic and Social Considerations
Biomass energy, leveraging organic materials for power, offers an array of economic benefits that extend beyond its environmental advantages. These benefits span from enhancing energy independence and stimulating more green jobs, to revitalizing agricultural sectors and improving the quality of life in communities. Here are some key economic and social impacts associated with the deployment of biomass as an energy source:
- Contributes to energy independence by reducing reliance on imported fuels, lessening vulnerability to global market fluctuations.
- Stimulates job creation in rural areas with abundant biomass resources, with roles in harvesting, transportation, processing of biomass materials, and construction and operation of conversion facilities.
- Circulates money within the local economy and can decrease overall energy costs compared to traditional fossil fuels.
- Provides economic predictability for businesses due to a stable and potentially lower-cost energy supply.
- Creates new revenue streams for governments through taxes and tariffs from the biomass energy sector, which can be reinvested in public services or infrastructure.
- Revives underutilized agricultural sectors by providing an alternative market for crops and waste products, diversifying farm income and stabilizing agricultural markets.
- Integrates biomass production into existing farming practices, creating synergies like using agricultural waste for energy production.
- Improves quality of life by supplying local, renewable energy, and can be linked with educational and skills training programs, enhancing workforce expertise.
- Ensures benefits such as improved energy access and job creation are directly experienced at the community level due to the local nature of biomass resources.
Challenges Associated with Biomass Energy
Despite these benefits, the biomass energy sector confronts a suite of challenges that are environmental, social and economic in nature. For instance, while biomass energy can stimulate rural economies, there may be competition for land between bioenergy crops and food production, which could influence food prices and security, particularly in regions already susceptible to these pressures. Additionally, the significant initial capital required to establish biomass conversion facilities can be a formidable barrier, particularly in developing economies.
The socioeconomic impacts also merit examination, especially in relation to land ownership and the fair distribution of economic benefits. In scenarios where large-scale biomass plantations are developed, there is a risk of marginalizing small landholders or impacting indigenous communities. This could lead to socioeconomic tensions if not managed with a comprehensive understanding of local dynamics.
Moreover, there can be a misconception that biomass resources are limitless, which can lead to unsustainable harvesting practices that harm ecosystems and biodiversity. It is therefore imperative to implement stringent resource management practices to ensure the environmental viability of biomass operations. Neglecting these practices could precipitate ecological imbalance and potentially diminish public support for the sector.
Such concerns underscore the need for a nuanced approach to incorporating biomass energy into the broader energy matrix. This approach must align with principles of ecological sustainability, social equity, and long-term economic viability to ensure that biomass energy can fulfill its promise as a sustainable and socially responsible energy source. Robust governance structures, transparent policies, and community engagement are critical components in realizing the potential while addressing the complex challenges associated with biomass energy.
Technological Innovations in Biomass Energy
Recent Technological Advancements in Biomass Energy Efficiency and Sustainability
Technological innovations are critical to amplifying the efficiency and sustainability of biomass energy systems. These advancements play a pivotal role in both optimizing energy production from biomass and minimizing its environmental footprint.
High-Yield Energy Crops
One essential breakthrough has been the development of high-yield energy crops. These crops are selectively bred or genetically modified to produce more biomass per acre, enhancing the raw material’s availability for energy production without expanding land use excessively.
Advancements in Pre-treatment Processes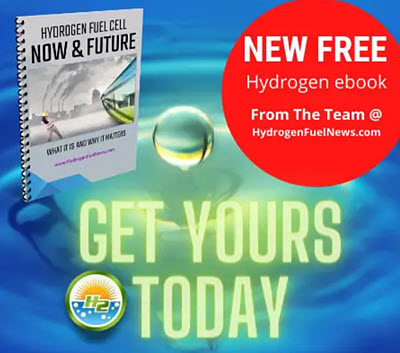
Additionally, advancements in pre-treatment processes enable the more effective breakdown of biomass materials. By using enzymes or other biochemical agents, lignocellulosic biomass—plant materials composed primarily of cellulose, hemicellulose, and lignin—is more efficiently converted into biofuels. This advancement improves bioenergy yields while reducing the amount of energy input required for conversion, thereby increasing overall sustainability.
Microorganism Engineering
Scientists and engineers are also exploring microorganism engineering, an avenue where specific bacteria and fungi are modified to boost biogas production rates. Through metabolically engineered microorganisms, both the speed and volume of biogas output from anaerobic digestion processes can be increased, thereby optimizing energy generation from waste biomass.
Co-firing Technologies
Co-firing technologies represent another significant advancement. Utilizing biomass in conjunction with coal in existing power plants reduces reliance on fossil fuels. The efficiency of co-firing systems is enhanced through advancements in feedstock handling, storage, and combustion, enabling a smoother integration of biomass in high-energy processes.
Improvements in Biomass-to-Liquid (BtL) Conversion
Further, improvements in biomass-to-liquid (BtL) conversion technologies are promising for the transportation sector. These techniques involve transforming solid biomass into synthetic fuels that can replace diesel or gasoline. Advancements here center on catalysis and process intensification, ensuring the conversion processes are more energy-efficient and result in fuels that are cleaner-burning and more compatible with current engines.
Integration of Smart Technology
Moreover, the integration of smart technology into biomass production and energy conversion processes is a burgeoning area of interest. Through precision agriculture techniques, the cultivation of biomass can be more efficiently managed, resulting in less waste and higher-quality energy crops. Smart sensors and IoT (Internet of Things) devices monitor and optimize the conditions of biomass storage and conversion processes, leading to higher process stability and efficiency.
Utilization of Heat Generated During Processing
Capturing the heat generated during biomass processing for use in district heating systems represents another key development. By utilizing this produced thermal energy, the overall efficiency of the biomass conversion process is elevated, contributing to a reduction in energy waste.
Integration with Other Renewable Sources
Lastly, there is growing interest in integrating biomass energy systems with other renewable sources, such as solar and wind. These hybrid systems can potentially lead to more stable energy output, addressing the intermittent nature of some renewable energy sources and contributing to a more resilient energy grid.
It is evident that each technological stride not only brings us closer to a more energy-secure future but also reinforces biomass energy’s role within a diversified and sustainable energy portfolio. Researchers are continuously exploring further innovative means to improve biomass fuel quality, conversion processes, and environmental impacts, grounding their commitment to the valorization of biomass as a cornerstone of renewable energy narratives.
Policy Framework and Future Outlook
Current policies greatly influence the adoption rate and scaling of biomass energy technologies. For example, subsidies, tax incentives, and grants can make biomass projects more economically feasible, incentivizing industries and communities to invest in this renewable energy source. Conversely, stringent environmental regulations can rightfully impose limits on emissions from biomass energy production but may also increase operating costs, thus affecting its competitive edge in the market.
One of the principal policy tools is the Renewable Portfolio Standard (RPS), which mandates that a certain percentage of electricity must be generated from renewable sources. Such policies nudge utilities to incorporate biomass into their energy mix. Furthermore, feed-in tariffs guarantee a fixed premium price for biomass electricity, ensuring revenue stability for biomass plants.
The future developments in the biomass energy sector will likely depend on continuous policy evolution. Nations are drafting more tailored legislation that promotes sustainable biomass sourcing and production methods to mitigate negative ecological impacts and support local economies.
Innovation in technology and practices is expected to continue, leading to more efficient and cleaner biomass energy production systems. Second and third-generation biofuels, which are derived from non-food biomass sources, are set to play a significant role in reducing competition with food supplies. Investment and research into these areas signal a shift towards more sustainable fuel alternatives.
Policies that support community-scale biomass systems are also gaining traction. These systems can provide local jobs, bolster energy independence, and encourage community participation in renewable energy adoption. Additionally, by focusing on local sourcing and minimal transportation, these small-scale systems can significantly reduce the carbon footprint associated with biomass energy.
Educational programs and skills training can expand as policies increasingly recognize the social value of equipping individuals with the knowledge to participate in the bio-economy. As the sector grows, a skilled workforce will be essential for it to thrive.
With international climate goals becoming more urgent, policies may increasingly incorporate the biomass sector into broader climate strategies. This includes potentially linking biomass production with carbon pricing mechanisms, providing financial motivation to reduce emissions.

In conclusion, biomass energy presents a compelling case as an integral part of a sustainable and diversified energy portfolio. It offers numerous benefits such as contributing to energy independence, stimulating job creation, and providing a renewable source of energy, thereby playing a significant role in the transition towards a low-carbon economy.
Technological advancements continue to enhance its efficiency and sustainability, making it a more viable alternative to fossil fuels. However, it is important to acknowledge that biomass energy also has its cons, including the potential for deforestation and the emission of greenhouse gases if not properly managed. Therefore, it is essential to ensure responsible sourcing and use of biomass materials and continue improving technology and practices to mitigate these challenges. With the right balance, biomass energy can certainly be a key player in our future energy landscape.