No, White Hydrogen Isn’t A Limitless Source Of Clean Fuel
Recently people have been asking me to provide an opinion on a new color of hydrogen, white. My color blindness makes the entire hydrogen color spectrum even sillier than it already is, as I think we’re at 23 more colors than required at present.
Really, there are only two types of hydrogen — low-carbon hydrogen and high-carbon hydrogen. If colors are applied, they should be green and black. The Hydrogen Science Coalition founders, engineers with long professional and academic experience of hydrogen, argued among themselves about what a climate-solution aligned cutoff point should be for hydrogen manufacturing, and settled on a kilogram of carbon dioxide or equivalent (CO2e) generated in manufacturing a kilogram of hydrogen. That pretty good threshold suggests a kilogram or less is green, and everything else is black. I’d be okay with that. At least I could tell the colors apart.
Full lifecycle carbon intensity of hydrogen is the point, not the process or raw materials used to manufacture it. All of the colors are just variants of process or source, which is causing headaches even among people with normal color perception, not to mention competing definitions of colors.
Let’s examine what one kilogram per kilogram carbon intensity means, just a little bit. It takes, with balance of plant, 55 to 60 kWh of electricity to manufacture a kilogram of hydrogen using electrolysis. That’s not going to become magically smaller, by the way, as we’re approaching the limits of physics on this point. It’s not susceptible to Wright’s law, where a doubling of units manufactured reduces costs per unit by 20% to 27%. There are scaling economies available to hydrogen manufacturing, but mostly by making very big plants, which will still be very capital intensive.
Using the one kilogram of CO2e, that means that each kWh of electricity can have a carbon debt of about 18 grams of CO2e. That works just fine in my home province of British Columbia in Canada from grid electricity, which has a carbon intensity of 12.9 grams CO2e per kWh. Vermont has an even lower level of CO2e per kWh, and could manufacture green hydrogen by this definition from grid electricity, while even Washington State can’t cut it at about 84 grams CO2e/kWh. Over in Europe, Sweden comes closest, but is still at 45 grams CO2e/kWh, over double the cutoff. And all grid electricity is decarbonizing, albeit not as quickly as required. Eventually all grids will be in the British Columbia and Vermont range.
Still, I’d rather have grid hydrogen in Vermont or Sweden than hydrogen manufactured from natural gas, which between upstream methane emissions and the steam reformation process is in the 10 kilograms of CO2e per kilogram of hydrogen. And blue hydrogen is likely to only bring that down to 2-4 kilograms of CO2e per kilogram of hydrogen, and the lower end of that range requires very stringent upstream methane emissions management.
For context on the degree of lobbying around what constitutes ‘green’ hydrogen, the EU rules for renewable hydrogen peg carbon intensity that’s good enough at 3.38 kilograms of CO2e per kilogram of hydrogen. Yes, the Hydrogen Science Coalition thinks that’s not a good level. Yes, there’s a lot of criticism of that carbon intensity from a wide variety of sources. There’s a good reason for that. It’s not remotely aligned with hitting climate goals, even if the EU wasn’t foolishly and temporarily obsessed with making hydrogen a carrier of energy. But it is within reach for hydrogen manufactured from natural gas. Convenient, no?
Why am I so focused on grid electricity? Two reasons.
The first is that 85% of hydrogen we use today is manufactured at point-of-use. That’s because hydrogen is so expensive to distribute. Most hydrogen fuel cell vehicle pumps are dispensing black hydrogen made from fossil fuels. That’s the cheapest form of hydrogen we’ve had to date. In the USA, it’s possible to manufacture hydrogen from dirt cheap natural gas for just under one US dollar per kilogram. Despite that, a truck load of hydrogen delivered is usually US$10 per kilogram. And a kilogram of hydrogen dispensed at a fuel cell pump has varied between US$15-25 over the past few years.
It takes about 14 tube trucks of hydrogen to deliver the same energy content as a single tanker truck of gasoline. That’s 14 times the distance traveled at minimum. 14 times the duration of paying a driver. 14 times the maintenance on trucks. And those tube trucks have compressed hydrogen or liquid hydrogen, both of which are energy intensive to compress or liquify. At the fuel cell pumping station, the storage tanks have to be compressed a lot. And if liquid hydrogen is being delivered, a bunch of tech is required to get it back into a gas quickly and safely. And then when it’s pumped into cars, a pump capable of compressing the gas to 700 bar (10,000 pounds per square inch), or about 700 times the pressure of the atmosphere at sea level is required. By comparison, scuba tanks top out at 300 bar.
Distribution of hydrogen is challenging, expensive, and risk prone. So we only do it today if we have to. Remember, diesel and gasoline are liquids at normal temperatures, and natural gas doesn’t have to be compressed much to hold sufficient energy to be worthwhile. We distribute so much of those fossil fuels in part because they are so easy and cheap to distribute.
The next part is cost. Electrolyzing hydrogen is a capex vs opex optimization game. Capex, or capital expenditure, is the cost to build the facility, and it has to be amortized over the kilograms of hydrogen produced. That means that a high capex drives a requirement for high utilization. That drives a requirement for electricity that’s available 60%+ of the year, or firmed electricity. You can’t get that with a single wind farm or a single solar farm, and if you build either to manufacture hydrogen you have to add those capital costs into the capex mix too. You are unlikely to get that with a wind farm and solar farm combined, either, even if you remarkably have good conditions for both wind and solar on the same site. At minimum you have to add some storage or transmission or both, and that increases the capex too. Oh, and you have to have a lot more operational expenses bolted on to manage all of the above, so your opex goes up, and you need close to grid management levels of expertise.
Of the components of an industrial scale electrolysis facility, only the electrolyzer itself has room for reductions in cost due to manufacturing volumes. The rest of the components are relatively commoditized industrial components, and there are 27 or so of them. Electrolysis plant costs aren’t going to be cut by 90%, and probably by no more than 20% over time.
On the other side of the equation, we have a great source of firmed electricity today. It’s call the grid. We connect multi-MW demand sources to the grid every day. It takes some planning, but it’s a lot cheaper from a capex perspective than building a wind and solar farm in the middle of nowhere.
But firmed electricity from the grid, which comes with the benefit of existing grid-competent operations, comes with utility administration costs and the like. That increases the opex side of the equation.
Build a facility in the middle of nowhere with wind, solar, storage, transmission, and an industrial electrolysis facility, and you have a very large capex with a smaller opex burden, but the huge distribution problem. Tie an existing ammonia facility to the grid, reuse the water supply for steam reformation, replace the steam reformation facility with electrolysis, and you have a much lower capital cost with higher operating costs, but without the distribution problem.
Building an appropriately-scaled hydrogen electrolysis facility at an ammonia plant and running it on grid electricity is going to be cheaper in most cases than delivering hydrogen manufactured a long way away to the ammonia plant, or building a net new ammonia plant in the middle of nowhere with an electrolysis facility. Put an electrolyzer at a nuclear plant to provide its hundreds of kilograms per day turbine lubricant habit is cheaper than shipping in black or gray hydrogen.
So my projection is that the vast majority of hydrogen manufacturing facilities are most reasonably placed at current demand points, especially ammonia manufacturing plants (the biggest persisting demand area for hydrogen), and supplied with grid electricity. There will be cases where centralizing massive electrolysis facilities at new build demand centers makes sense, but nowhere near the scale of current hype-driven proposals based on irrational cost costs and irrational demand projections.
Decarbonize the grid, manufacture hydrogen where it’s needed from grid electricity. As Hydrogen Science Coalition co-founder Paul Martin and I discussed recently, it’s completely reasonable to have strong additionality, locality, and temporality requirements for any federal subsidies, such as those from the US IRA. That means that purported green hydrogen facilities need to add renewable electricity that’s pretty close on the grid to the hydrogen facility, and manufacture electricity that’s entering the grid on a similar schedule to electricity demand from the hydrogen facility. Where they aren’t getting a subsidy, grid electricity will be a better and better choice in any event.
But in countries that apply an increasing carbon price, some of that starts to become a wash as long as we measure the CO2e emissions along the way and apply the carbon price to them. Canada’s carbon price, for example, includes methane, a key global warming gas. Grid electricity gets hit with carbon pricing, which is part of the reason why Alberta’s coal plants shut down early, decarbonizing their electricity quite rapidly. The EU emissions trading scheme (ETS) is inclusive of more and more things, increasing in price per ton and their carbon border adjustment mechanism is based on the ETS, so everything exported to the EU effectively has the the EU’s carbon price. And while the ETS does not include methane today, it will include it in 2026.
Okay, so it’s going to make sense to manufacture hydrogen locally more than not, and hydrogen isn’t going to be cheap.
Enter white hydrogen. What’s that? It’s naturally occurring deposits of gaseous hydrogen underground, hence sometime being called natural hydrogen. Same principle as natural gas (note the naming similarity) or oil or coal. Some mixture of biological and geological processes leads to there being hydrogen underground that might be able to be extracted.
Breathless headlines are all over it. Claims of limitless hydrogen abound. The hydrogen advocates who are increasingly dismayed by spreadsheet jockeys using realistic numbers finding that hydrogen doesn’t make sense as a store of energy are jumping onto the white hydrogen bandwagon.
But the enthusiasm is unwarranted.
The biggest such announcement recently came out of France in the Lorraine region, which, while better known for wine, was also a coal mining center. They have provisionally estimated that a deposit could have 46 million tons of hydrogen. That sounds like a lot. But we use about 120 million tons of hydrogen annually today, either in pure form or as synthetic gas (syngas), so it’s under 40% of a year’s demand.
So what, if we find a lot of deposits, right? Well, another site was found in Spain. How much hydrogen does it have? Well, if it were all hydrogen, it would be about 1.2 million tons, or about 1% of a year’s demand. And it isn’t, as it’s mixed with other gases. Another site had half a day’s current demand of hydrogen in its estimated reserves.
Well, that’s fine. We can just pump it out and use it, right? Well, no. The French site has hydrogen that’s dissolved in an underground aquifer of ‘liquid’. It’s about 16% hydrogen at a kilometer underground, and increases to approaching 98% hydrogen at 3 kilometers underground per the researchers. (How exactly hydrogen concentrations increase radically at the bottom of an aquifer of liquid is unexplained, and I’m at a bit of a loss. If anyone knows the mechanism by which this would occur, please enlighten me.)
What exactly is the liquid? What else is in the liquid? What is the process by which the hydrogen is extracted from the liquid. What is the cost of this extraction? What other gases might escape from the aquifer during hydrogen extraction? All unanswered questions. We don’t extract hydrogen from underground at present, and so have at best provisional answers to the questions.
We already have a big well-head methane leakage problem at natural gas extraction sites, and methane is a much bigger and less leaky molecule than hydrogen. How much hydrogen will be lost to the atmosphere? What are the implications given that hydrogen has an indirect global warming potential over ten times that of carbon dioxide?
And the French researchers are careful to point out that their initial estimates are just that, and there’s a lot of work to confirm their numbers.
So, the biggest find so far is at most 40% of one year’s current hydrogen demand, and it’s unclear what it will cost to extract it. Oh, and it’s not terribly near demand centers. The nearest Yara (biggest ammonia manufacturer in Europe) plant in France is on the other side of the country, 700 kilometers away. There’s one in the Netherlands that’s under 400 km away. There is an oil refinery just across the border in Germany, the MiRO refinery 40 km away, but are we really going to waste white hydrogen on hydrodesulfurization of transportation fuels when that market is in steep decline and the world is going to be awash in low sulfur crude oil in the coming decades? Probably not.
In other words, even if the hydrogen can be extracted reasonably cheaply, perhaps as cheaply as manufacturing it from natural gas, it’s still not at points of demand. Hydrogen refueling stations on highways won’t be able to drill a hole a few hundred meters deep and fill up their hydrogen storage tanks. It might make sense if it can be extracted at a reasonable price to build a facility on top of the site that will drain it over 30 years, or it might not. I’ll leave that to the spreadsheet jockeys.
Chip in a few dollars a month to help support independent cleantech coverage that helps to accelerate the cleantech revolution!
There is a place in Africa, a village in the landlocked Republic of Mali in western Saharan Africa, that actually has 98% pure hydrogen that comes out of the ground, which they burn to make electricity in a little turbine. That supplies the town of 4,000 people. Not really the basis for a global economy, even though there is more underground. And Mali is, once again, a long way from demand centers for hydrogen.
So there’s hydrogen underground, more than we previously thought. It’s concentrated in some areas in volumes vastly lower than current global hydrogen demand. It’s typically a long way from hydrogen demand centers. We don’t know how much it will cost to extract and process, but we do know that it will cost a lot to distribute. It’s not the basis of a radical expansion of a hydrogen for energy economy, but does hold out some hope for decarbonization of some of current hydrogen use.
After all, hydrogen is currently a global warming emissions problem on the scale of all of aviation.
The Lorraine find should be explored and exploited if possible. Among other things, it’s competing with US$1-3 per kilogram black hydrogen from natural gas. Assuming even a buck a kilogram, that’s potential revenue of US$46 billion — well worth someone’s effort to develop and exploit. But it’s not a reason to waste hydrogen on transportation or heating.
Have a tip for CleanTechnica? Want to advertise? Want to suggest a guest for our CleanTech Talk podcast? Contact us here.
Latest CleanTechnica.TV Video
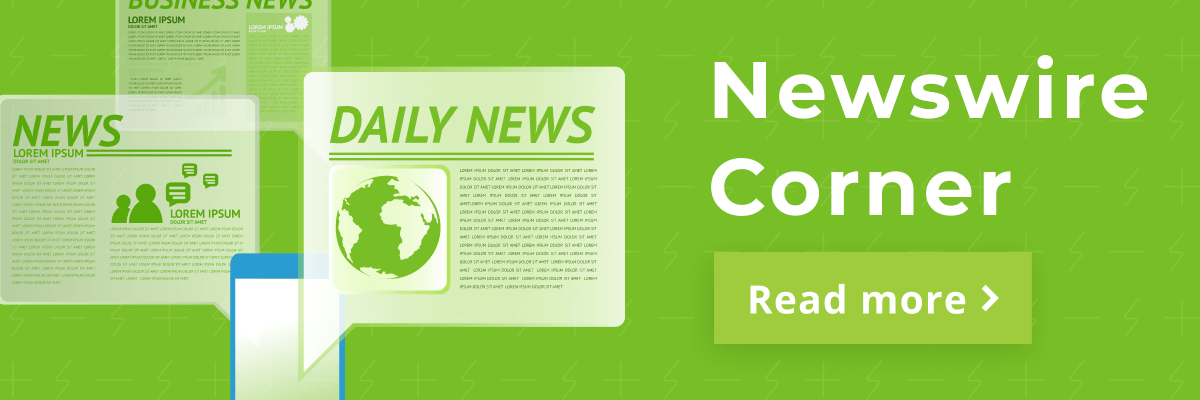
CleanTechnica uses affiliate links. See our policy here.